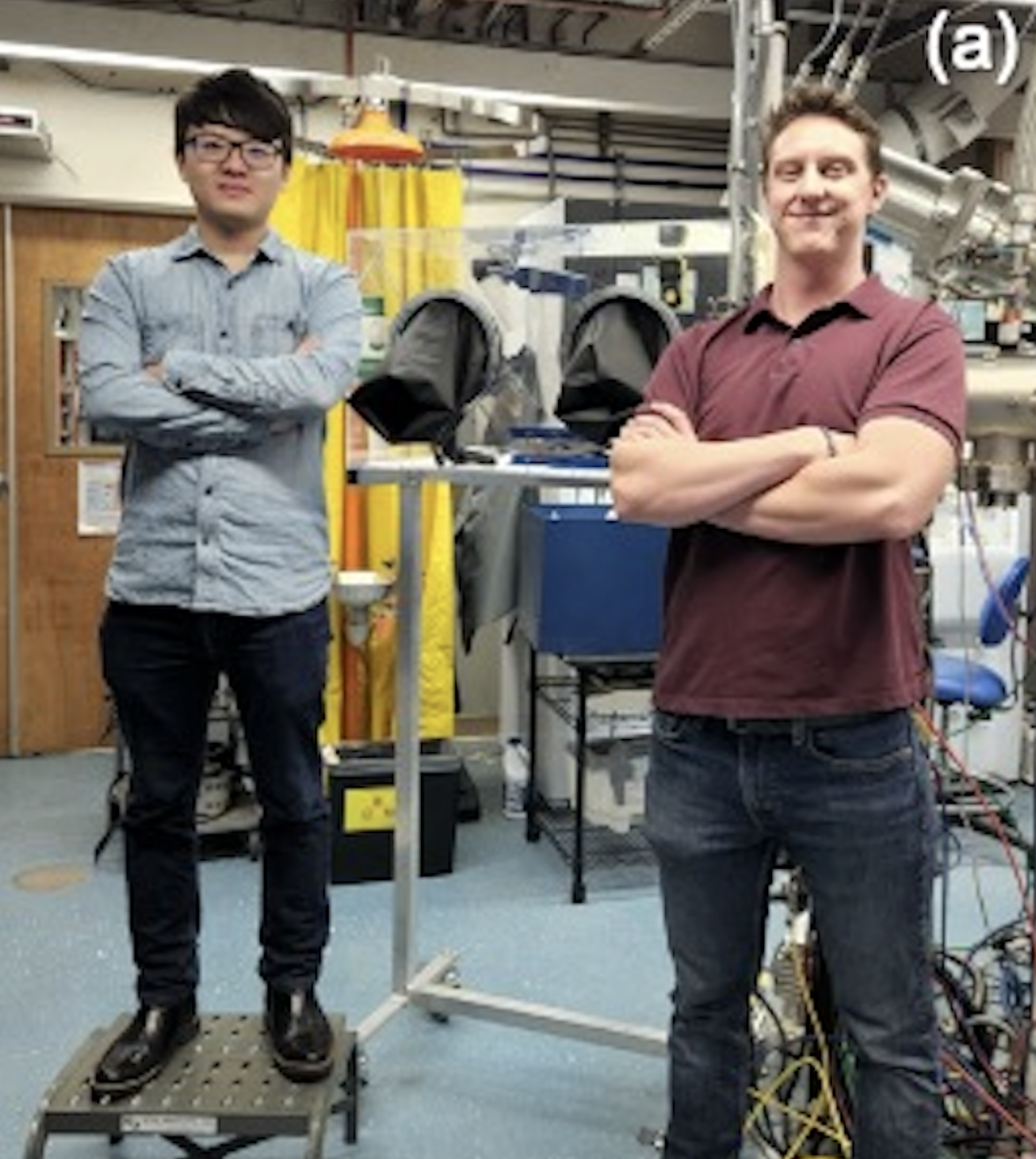
Molecular Beam Epitaxy of Candidate Topological Superconductor Sr3SnO
Sr3SnO is a novel antiperovskite material that is a candidate intrinsic topological superconductor. Intrinsic topological superconductors, similar to the proximitized topological superconductors posited by Fu and Kane [1], are predicted to host Majorana fermion states on their edges. Majorana fermions are their own antiparticle, and they obey what is known as non-Abelian exchange statistics [2]. These non-Abelian statistics, as well as protection against many forms of decoherence, make Majorana fermions interesting for the possibility of future low-decoherence topological quantum computing [3]. Sr3SnO is predicted to be an intrinsic topological superconductor due to the combination of two observed properties: (1) a topologically nontrivial band structure, which identifies the material as a 3D Dirac semimetal [4–8]; and (2) superconductivity which has been observed in Sr-deficient polycrystalline samples (technically Sr3-xSnO) [9,10]. The co-existence of these properties could possibly point to a topological superconducting phase, though conclusive evidence for this has not yet been achieved and thus further study of high-quality samples is warranted. Indeed, though a number of different materials have been posited to be intrinsic topological superconductors throughout the years, there has still been no positive proof of such a novel phase. Additionally, the realization of an oxide 3D Dirac semimetal would in itself be an interesting phase to explore for possible high-mobility devices.
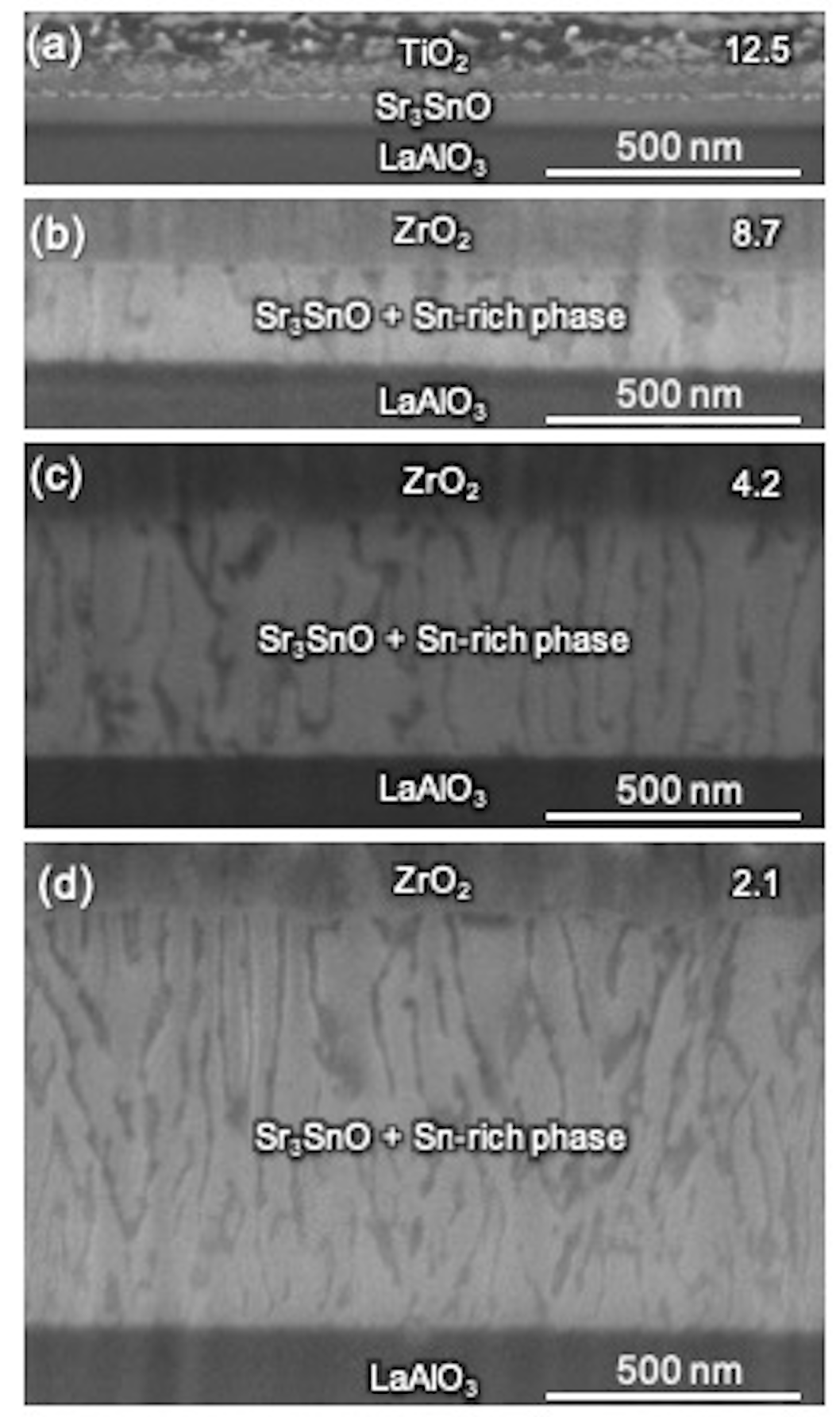
Growing Sr3SnO (and Sr3-xSnO) as a single-crystalline thin film, as opposed to bulk single crystals or polycrystals, has many advantages for electrical characterization due to the well-defined transport geometry, as well as the direct applicability to devices. A well-suited tool for growing films of this material is molecular beam epitaxy (MBE). MBE uses atomic or molecular beams emanating from high-purity source materials to grow high-quality (i.e. low defect density) films with extremely high precision and control. Sr3SnO and related antiperovskites are highly air-sensitive, and so performing ex situ measurements on them is not trivial. One technique to mitigate this is to deposit a protective capping layer in situ before the film is removed from the MBE chamber. This allows for x-ray diffraction (XRD) measurements – which check the atomic structure of the phases of the film – and scanning electron microscopy (SEM) measurements – which provide information on film morphology. Cross-section SEM images have shown that some films possessed multiple phases, seen as light and dark regions in Figure 1, though the secondary phase was not positively identified by XRD. By adjusting the MBE growth conditions (flux ratio in this case), phase-pure films could be achieved (Fig. 1) [11]. For electrical measurements, instead of depositing an in situ cap, films are removed from the MBE chamber directly into a glovebox that has been purged with high-purity N2 (Fig 2a). This allows the sample to be assembled onto a PPMS puck for electrical measurements while minimizing degradation of the film. Indium contacts are mechanically placed on four corners of the sample, and the remaining exposed surface of the sample is covered in vacuum grease for protection from air exposure when the sample is being transferred to the measurement system (Fig. 2b). Electrical characterization of films of Sr3SnO (Sr3-xSnO) is ongoing, but early measurements indicate that some of the expected electronic properties of a 3D Dirac semimetal are indeed realized [11,12], though superconductivity has remained elusive in thin films up to this point.
References
[1] L. Fu and C. L. Kane, Superconducting Proximity Effect and Majorana Fermions at the Surface of a Topological Insulator, Phys. Rev. Lett. 100, 1 (2008). [
2] F. Wilczek, Majorana Returns, Nat. Phys. 5, 614 (2009).
[3] M. Leijnse and K. Flensberg, Introduction to Topological Superconductivity and Majorana Fermions, Semicond. Sci. Technol. 27, 1 (2012).
[4] T. Kariyado and M. Ogata, Three-Dimensional Dirac Electrons at the Fermi Energy in Cubic Inverse Perovskites: Ca3PbO and Its Family, J. Phys. Soc. Japan 80, 1 (2011).
[5] T. H. Hsieh, J. Liu, and L. Fu, Topological Crystalline Insulators and Dirac Octets in Antiperovskites, Phys. Rev. B - Condens. Matter Mater. Phys. 90, 081112 (2014).
[6] A. Ikeda, T. Fukumoto, M. Oudah, J. N. Hausmann, S. Yonezawa, S. Kobayashi, M. Sato, C. Tassel, F. Takeiri, H. Takatsu, H. Kageyama, and Y. Maeno, Theoretical Band Structure of the Superconducting Antiperovskite Oxide Sr3−xSnO, Phys. B Condens. Matter 536, 752 (2018).
[7] T. Kawakami, T. Okamura, S. Kobayashi, and M. Sato, Topological Crystalline Materials of J=3 /2 Electrons: Antiperovskites, Dirac Points, and High Winding Topological Superconductivity, Phys. Rev. X 8, 1 (2018).
[8] Y. Fang and J. Cano, Higher-Order Topological Insulators in Antiperovskites, Phys. Rev. B 101, 245110 (2020).
[9] M. Oudah, A. Ikeda, J. N. Hausmann, S. Yonezawa, T. Fukumoto, S. Kobayashi, M. Sato, and Y. Maeno, Superconductivity in the Antiperovskite Dirac-Metal Oxide Sr3-XSnO, Nat. Commun. 7, 3 (2016).
[10] M. Oudah, J. N. Hausmann, S. Kitao, A. Ikeda, S. Yonezawa, M. Seto, and Y. Maeno, Evolution of Superconductivity with Sr-Deficiency in Antiperovskite Oxide Sr3−xSnO, Sci. Rep. 9, 1831 (2019).
[11] W. Wu, N. G. Combs, and S. Stemmer, Molecular Beam Epitaxy of Phase-Pure Antiperovskite Sr 3 SnO Thin Films, Appl. Phys. Lett. 119, 161903 (2021).
[12] H. Nakamura, D. Huang, J. Merz, E. Khalaf, P. Ostrovsky, A. Yaresko, D. Samal, and H. Takagi, Robust Weak Antilocalization Due to Spin-Orbital Entanglement in Dirac Material Sr3SnO, Nat. Commun. 11, 1 (2020).