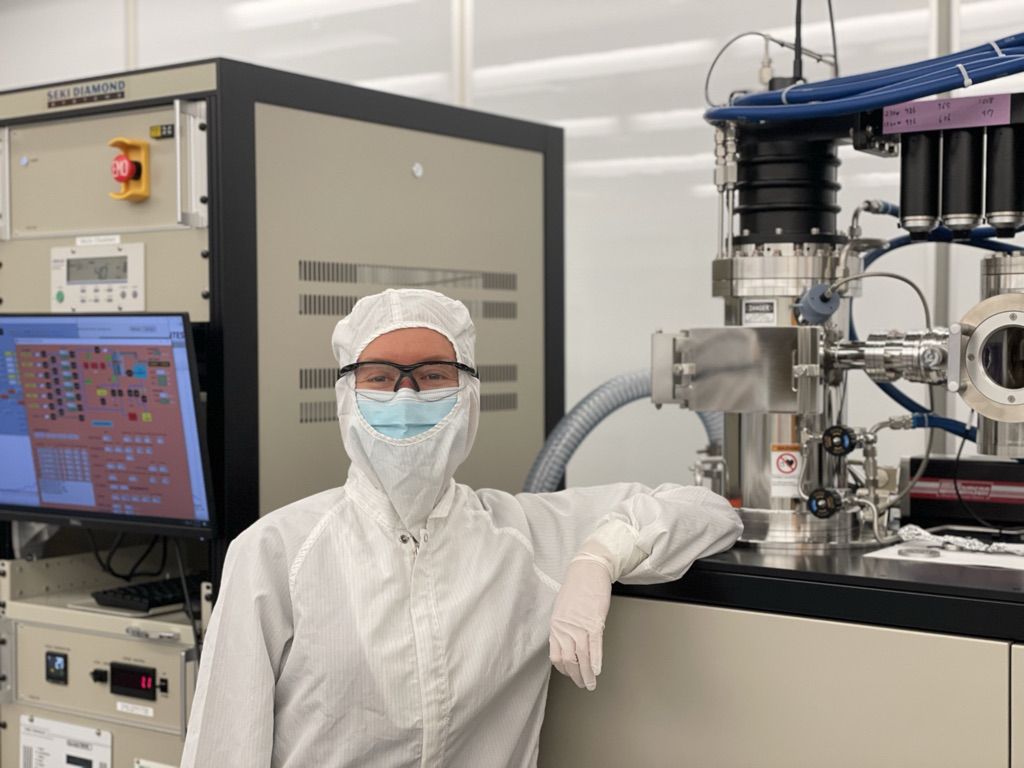
Two-Dimensional Spin Systems in PECVD-Grown Diamond with Tunable Density and Long Coherence for Enhanced Quantum Sensing and Simulation
Solid-state spins constitute a powerful platform for quantum technologies. Dense ensembles of coherent spins provide a starting point for investigating strongly interacting spin systems in which novel, many-body states can arise with applications in both quantum simulation and sensing. Dimensionality plays a critical role in the nature of many-body states, with reduced dimensionality giving access to unique phases and phenomena such as interaction-driven localization or dipolar-driven spin squeezing. For sensing applications, a dense 2D layer of sensors in close proximity to a sensing target exhibits enhanced spatial resolution (set by the depth of the layer) compared to a 3D ensemble at the same volumetric density, while benefiting from either 1/ÖN classical sensitivity enhancements or entanglement-driven enhancements. Lastly, dense 2D ensembles could serve as a starting point for targeted, on demand formation of individual, optically resolvable defects at specific locations, such as inside nanostructures. Altogether, creating thin spin layers with tunable density is of intense current interest but has been minimally explored in solid-state electronic spin systems to date.
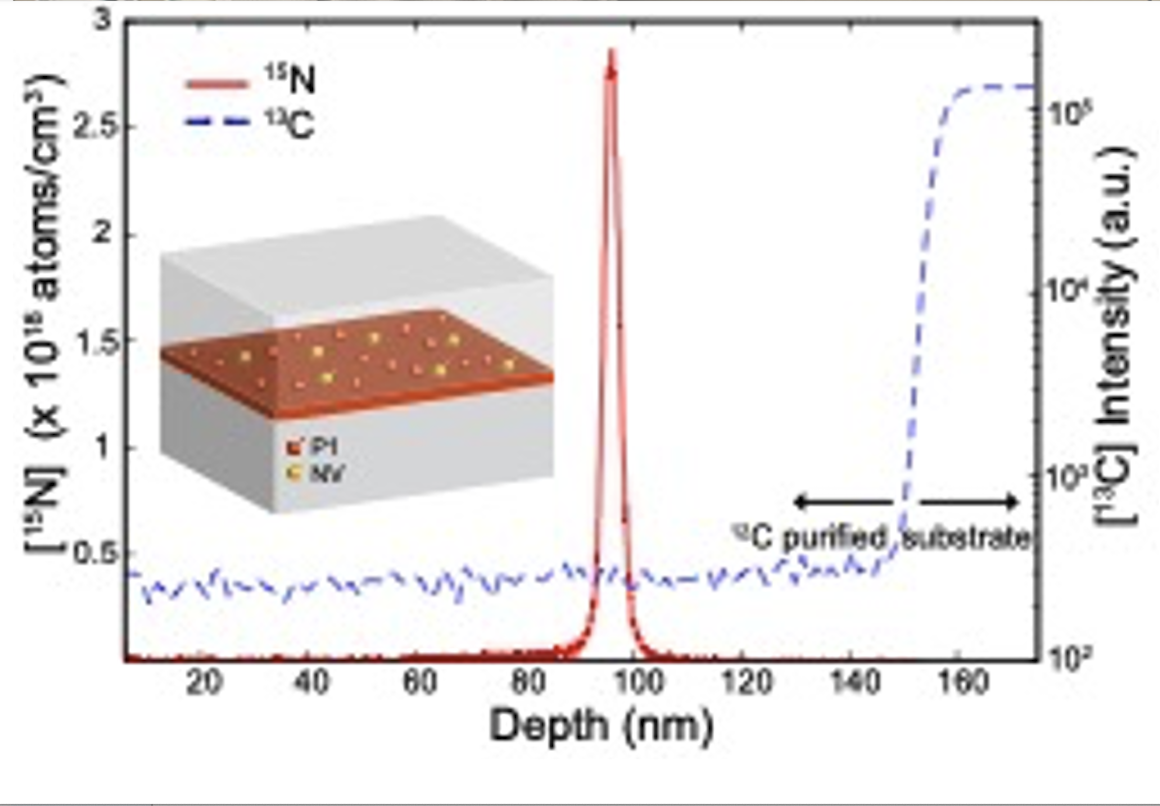
Defects in diamond, such as nitrogen-vacancy (NV) centers and substitutional nitrogen (P1 centers), are particularly promising solid-state platforms to explore. However, the ability to controllably create coherent, two-dimensional spin systems and characterize their properties, such as density, depth confinement, and coherence has been an outstanding materials challenge. We present a refined approach to engineer dense (³ 1 ppm·nm), 2D nitrogen and NV layers in (100) diamond using delta-doping during plasma-enhanced chemical vapor deposition (PECVD) epitaxial growth. We employ both traditional materials techniques, e.g., secondary ion mass spectrometry (SIMS), alongside NV spin decoherence-based measurements to characterize the density and dimensionality of the P1 and NV layers. We observe depth confinement of the spin layer down to < 4 nm, high (up to 0.74) ratios of NV to P1 centers, and reproducibly long NV coherence times which are dominated by dipolar interactions between the engineered P1 and NV spin baths. Altogether, the results of our joint materials and qubit-based approach are key elements in the engineering of solid-state-spin systems for the next generation of quantum technologies.1-2
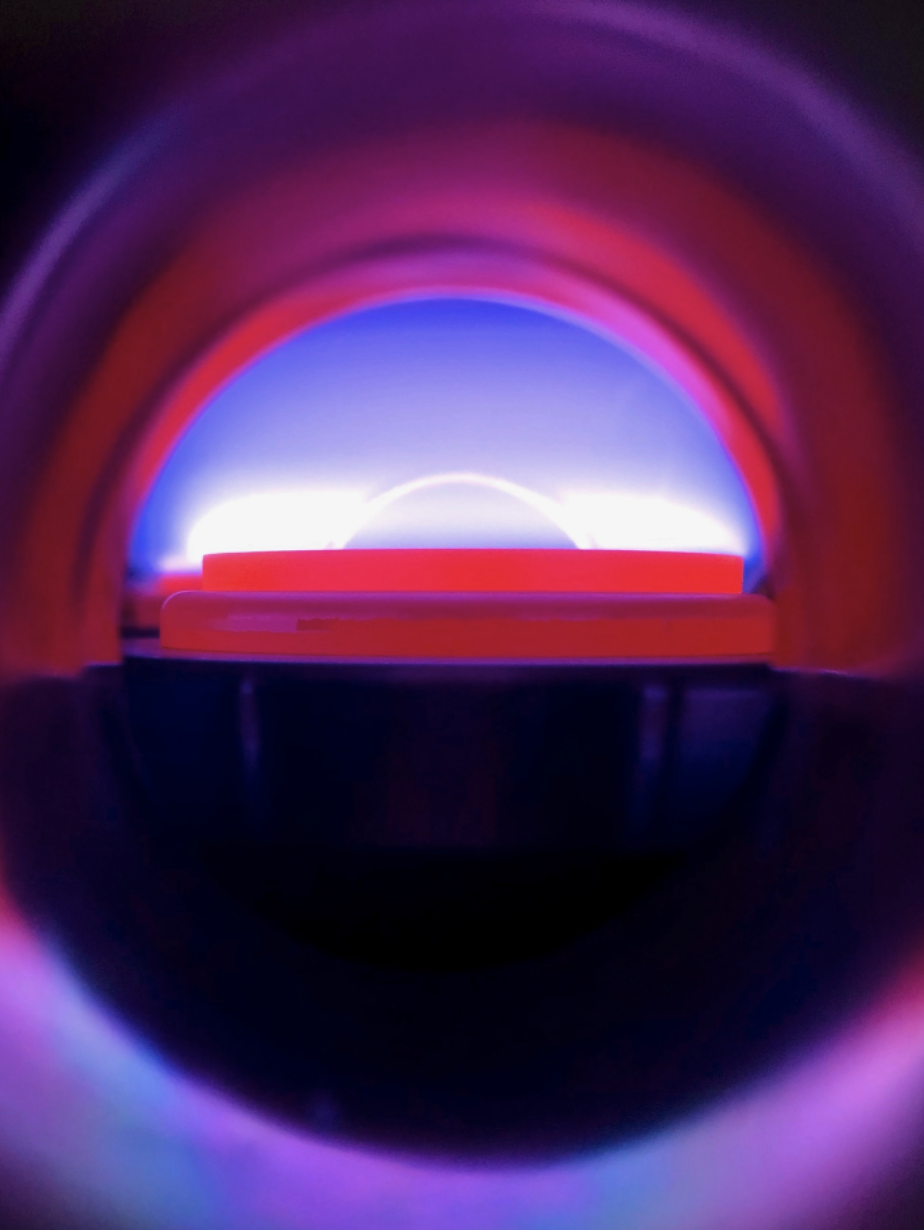
References
[1] L. B. Hughes, Z. Zhang, C. Jin, S. A. Meynell, B. Ye, W. Wu, Z. Wang, E. J. Davis, T. E. Mates, N. Yao, K. Mukherjee, A. C. B. Jayich, APL Mater. 11, 021101 (2023).
[2] E. Davis, B. Ye, F. Machado, S. Meynell, T. Mittiga, W. Schenken, M. Joos, B. Kobrin, Y. Lyu, D. Bluvstein, S. Choi, C. Zu, A. C. B. Jayich, and N. Y. Yao, Nat. Phys. (2023).